Janus particles are colloids with polar and apolar faces. The goal of this thrust is to test the efficacy of Janus particles as solid surfactants for emulsion stabilization. Because of the amphiphilicity of Janus particles, researchers in the field have suggested that these particles would be useful for emulsion stabilization. However, considering that emulsions can be readily stabilized with homogeneous particles to form the so-called Pickering emulsions, it is not evident that Janus particles would provide significant advantages over their counterparts (e.g., homogeneous particles) in stabilizing multiphasic fluid mixtures. We aim to understand, in depth, the behavior of Janus particles at an oil-water interface at the single particle level and to extend this understanding to the rheological properties of Janus particle monolayers at the fluid-fluid interfaces. Ultimately, the obtained understanding and knowledge will be correlated with studies designed to test the efficacy of Janus particles as solid surfactants to maintain emulsion stability.
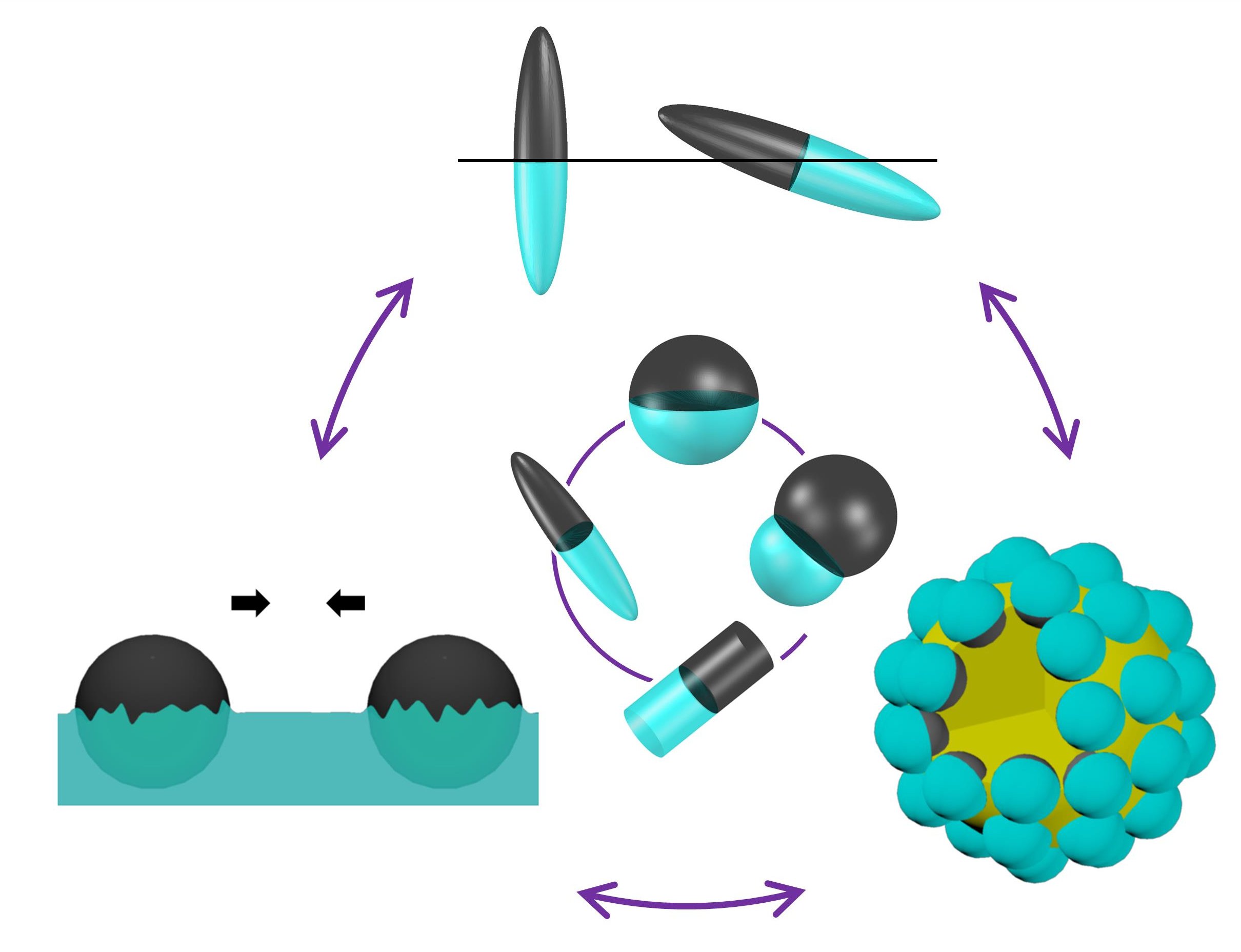
Projects
Emulsion Stabilization and Emulsions Phase Inversion Using Shape-Changing Janus Particles.It is well understood and accepted that the shape and chemistry of molecular surfactants govern their behaviors in solution. In this work, we seek to understand the effect of particle shape and surface chemistry on the behavior of Janus particles as solid surfactants. In particular, we synthesize shape-changing and amphiphilicity-reversing Janus dumbbells as solid surfactants to stabilize and invert emulsions. This work is motivated by our free energy calculations, which show that it is possible to generate thermodynamically stable emulsions and that the shape of Janus particles has an enormous impact on the size and type of emulsions that can be stabilized. We synthesize pH-responsive Janus dumbbells using seeded emulsion polymerization and show that these Janus particles can stabilize different types of emulsions depending on the solution pH of the aqueous phase. We also demonstrate, for the first time, the phase inversion of Janus particle-stabilized emulsions by pH-induced shape-change of Janus particles. In addition to simple emulsions, these Janus dumbbells allow for the generation of water-in-oil-in-water multiple emulsions in one emulsification step. These multiple emulsions can be induced to release the encapsulated materials upon changes in the solution pH.
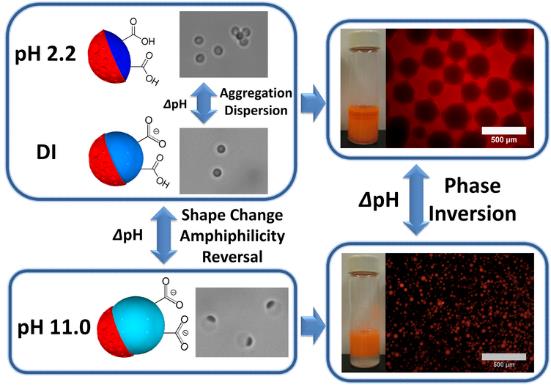
Non-spherical Janus Particles at Fluid Interfaces.The objective of this effort is to understand the effect of particle geometry on the orientation and interactions of Janus particles at fluid-fluid interfaces. We are currently investigating the equilibrium orientation of non-spherical Janus particles at an oil-water interface based on numerical calculations12. Two types of non-spherical Janus particles are considered: Janus ellipsoids and Janus dumbbells. We find that the equilibrium orientation of the interface trapped Janus particles strongly depends on the particle characteristics, such as their size, aspect ratio and surface properties (Figure 2). Interestingly, we find that non-spherical Janus particles, under appropriate conditions, can be kinetically trapped in a metastable state due to the presence of a secondary energy minimum. Our calculation, for the first time, provides a detailed guidance for the synthesis of non-spherical Janus particles, which can be used as effective solid surfactants for the stabilization of multiphasic fluid mixtures and the modification of the rheological properties of fluid interfaces. Our current efforts focus on generating particles with controlled anisotropy and surface properties to experimentally investigate their interfacial orientation.
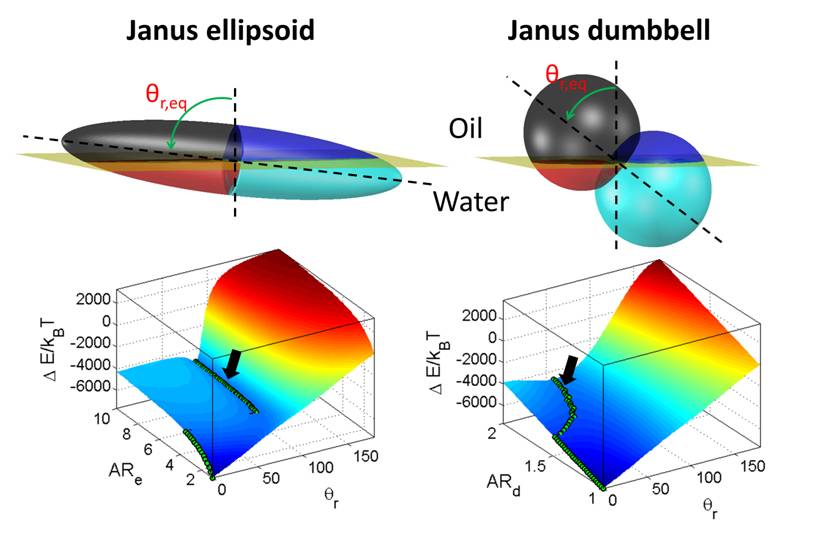
Janus Particles at Fluid-Fluid Interfaces. The goal of this study is to understand the behavior and assembly of chemically and geometrically asymmetric Janus particles at fluid-fluid interfaces. We have demonstrated that spherical Janus particles at interfaces interact with each other via attractive interactions. Such attractions are induced by the pinning of three-phase contact line around the diffuse boundary between the two sides of the particles. We are currently exploring the effect of patch shape and surface chemistry on the lateral interactions between particles.
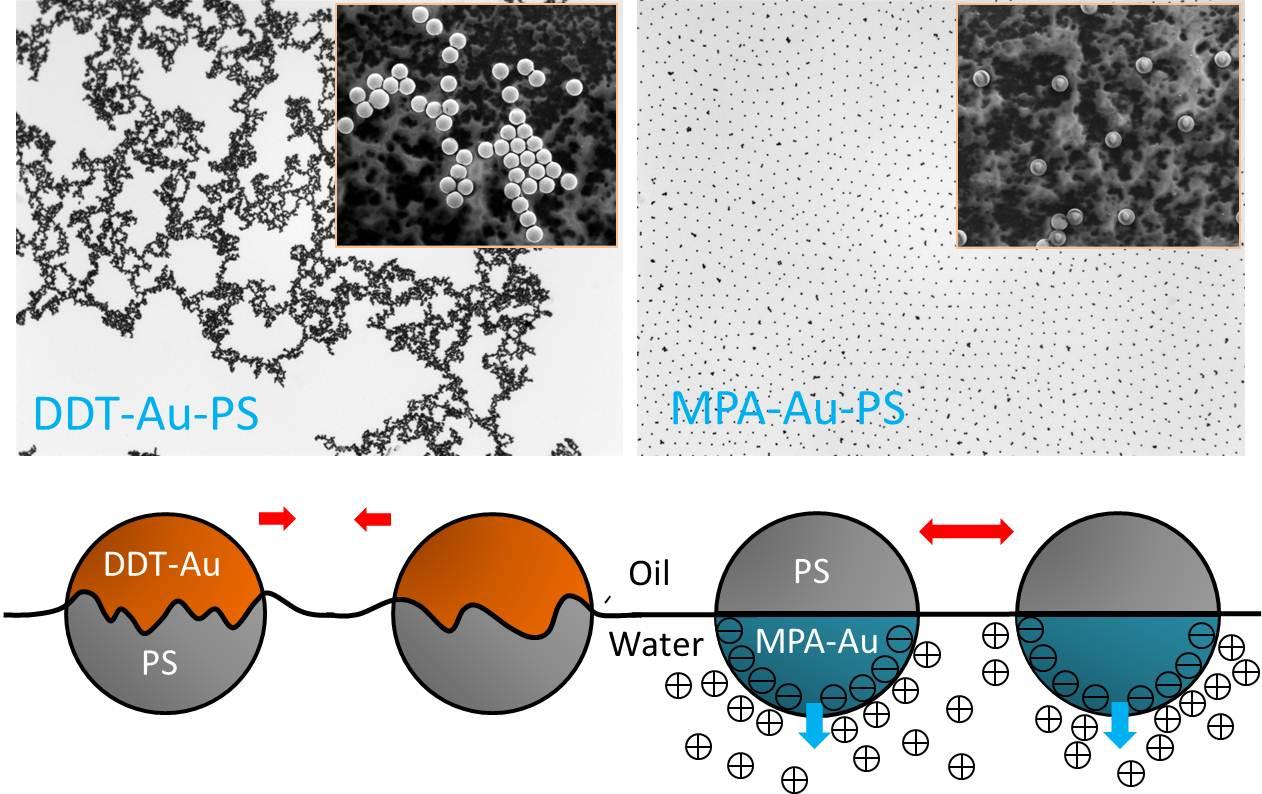
Assemblies of nanoparticles have shown great promises in providing synergistic and emergent properties that are useful for advanced applications ranging from water management to catalysis to sensing and energy conversion and storage. Despite countless demonstrations of useful functionality stemming from the combinations of various nanomaterials, very few examples of successful translation/commercialization of nanoparticle assemblies have thus far been achieved. We believe some of the key roadblocks that impede the widespread utilization of nanoparticle assemblies include 1) their rather poor mechanical robustness/durability and 2) the lack of scalable methods to manufacture such structures. One of the major thrusts in my group is to understand and establish the processing-structure-property relationship of nanoparticle assemblies using the state-of-the-art techniques and simulation-based methods with the goal of enhancing their mechanical robustness and durability. In addition, we are exploring scalable manufacturing of nanoparticle assemblies for applications in energy conversion & storage, separations and water management.
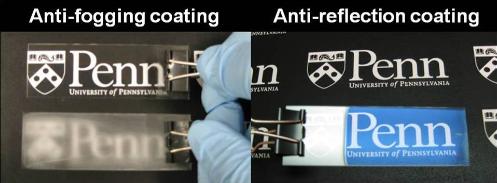
Projects
Nanocomposites with Extremely High Nanoparticle Loadings via Capillary Rise Infiltration (CaRI). Polymer nanocomposite films (PNCFs) combine the unique properties of nanomaterials with the toughness, flexibility and high processability of polymers, resulting in materials with synergistic properties and functionalities. Despite their utility in a number of advanced applications, PNCFs with high concentrations of nanoparticles are difficult to prepare because of their poor processability. We have recently developed a new method to prepare PNCFs with extremely high loadings of nanoparticles (volume fraction > 50%) by inducing the infiltration of a polymer into a film of densely packed nanoparticles. This method involves capillary rise infiltration (CaRI) of polymer into a dense packing of nanoparticles. In CaRI, a two-layer film, consisting of a porous layer of nanoparticles and a layer of polymer, is first prepared and subsequently this bilayer structure is annealed above the glass transition temperature of the polymer. Thermal annealing induces polymer infiltration into the interstices of the nanoparticle layer. The objective of this work is to understand the dynamics of polymer infiltration into nanoparticle films and to understand the processing-structure relationship of PNCFs generated based on this process.
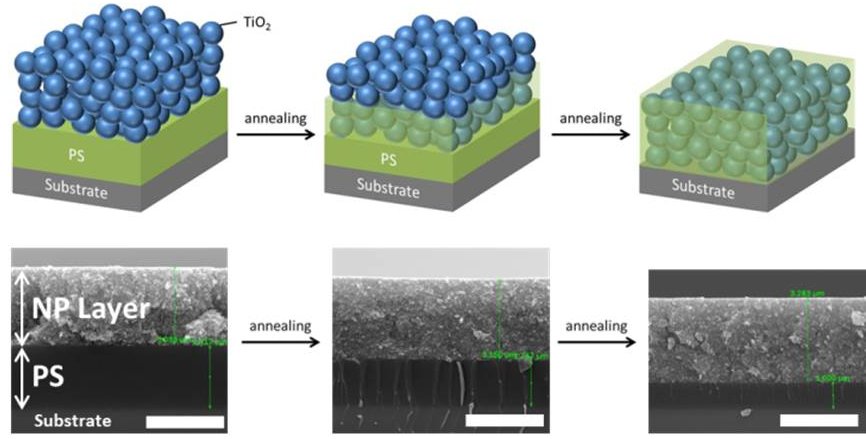
Mechanical Behavior of Disordered Nanoparticle Assemblies. Nanoparticle assemblies made of randomly packed particles are ubiquitous in energy storage and conversion devices as well as electronic displays, separation membranes and catalytic systems. One of the key drawbacks of these disordered nanoparticle assemblies is that they tend to be very brittle and have poor mechanical durability. The major goal of this effort is to understand the fundamental mechanisms that lead to the deformation and failure of these disordered nanoparticle assemblies (DNAs) and to explore new ways to toughen these structures. In assemblies with a well-ordered structure (i.e., lattice), the process of mechanical failure is controlled by defects in the packing, particularly by dislocations. There are, however, no obvious defects that control deformation and failure in disordered packings. The absence of clearly definable defects hinders deep understanding of the mechanical behavior of DNAs and makes it even more challenging to develop design rules to enhance their reliability and durability. One of the main hypotheses that form the basis of this work is that the mechanical behavior of DNAs are analogous to the mechanical response of other types of randomly structured (amorphous) materials such as metallic glasses and granular packings. Mechanical failure processes in dense, disordered packings of atoms such as in metallic glasses and of macroscopic grains as in granular media form extended shear bands, which are narrow zones of concentrated shear strain, which often lead to brittle fracture or other instabilities like stick-slip motion. Our recent study has indeed shown that DNAs fail by forming shear bands; however, we have also shown that particle anisotropy can toughen DNAs by suppressing shear band formation.
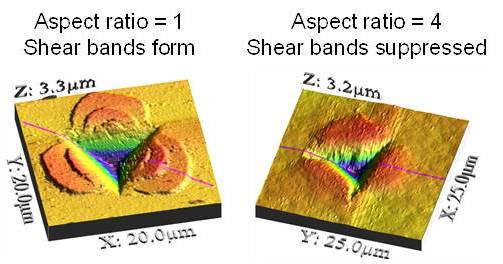
Avoiding Cracks in Nanoparticle Films. Cracks form in nanoparticle films during drying because capillary stress imposed by the suspending medium results in tensile stress in the plane of the film, leading to crack initiation. These cracks adversely affect the properties of NFs and limit their utilization in a variety of applications. A new method utilizing subsequent depositions of thin crack-free nanoparticle layers is demonstrated to avoid the formation of cracks within silica nanoparticle films. Using this method, crack-free films can be assembled with thicknesses exceeding the critical cracking values. Explanation of this observed phenomenon is hypothesized to mainly arise from chemical bond formation between neighboring silica nanoparticles. Application of this method for fabricating crack-free functional structures is demonstrated by producing crack-free Bragg reflectors that exhibit structural color. We are also exploring utilization of crack-free Bragg reflectors in enhancing the efficiency of the dye-sensitized solar cell.
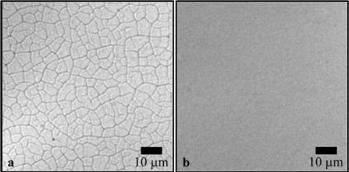
Mechanical Reinforcement of Nanoparticle Films using Atomic Layer Deposition. The objective of this project is to understand the effect of atomic layer deposition on the mechanical behavior of nanoparticle films. We are exploring atomic layer deposition (ALD) as a means to mechanically strengthen nanoparticle films (NFs) on a variety of substrates including polymers, and study how ALD fundamentally influences the mechanical characteristics and the failure mechanisms of nanoparticle films. This project is motivated by our recent success in imparting mechanical robustness to NFs on polymeric substrates. This project bridges the fundamental investigation of the nanostructure of ALD-treated NTFs to mechanical characteristics and failure mechanism of these films. We are studying the mechanical response of ALD-treated NFs under different loading geometry and length scale including nanoindentation, nanoscratching, bending and macroscopic abrasion.
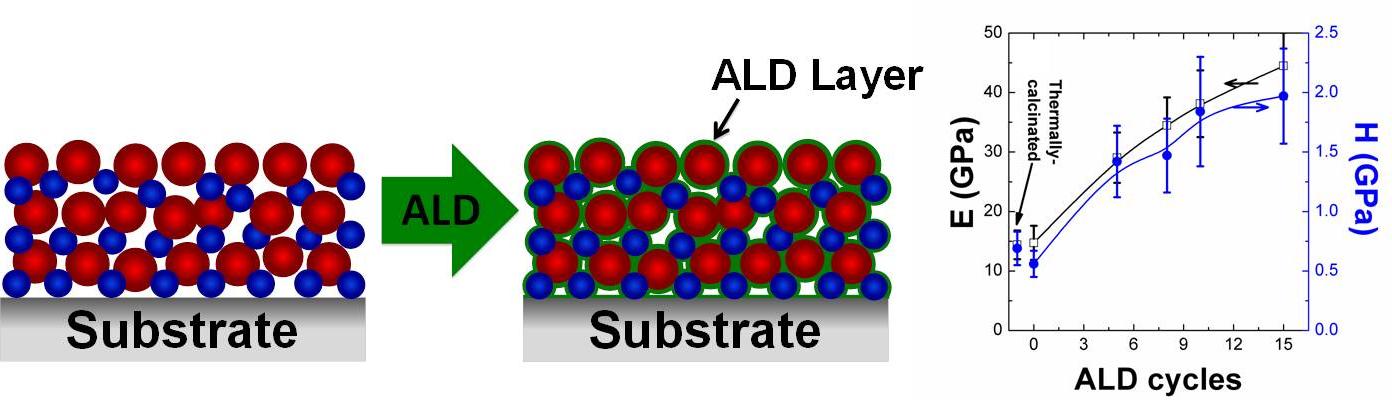
Electrostatic interactions in non-polar media for nanostructured thin film fabrication. The objective of this project is to understand a recently developed non-aqueous layer-by-layer assembly of nanostructured thin films by studying the fundamentals of electrostatic interactions in non-polar media. Layer-by-layer (LbL) assembly, which involves the sequential deposition of oppositely charged species, provides a simple yet versatile method for the generation of functional nanostructured thin films; however, this technique, to date, has been mainly used with water-soluble materials. This practice places a major obstacle to generating functional nanostructured thin films, because a large number of useful and unique nanomaterials are synthesized and stable in non-aqueous non-polar solvents only. The proposed activities are based on our recent success in imparting surface charge to various oxide and carbon-based nanomaterials in non-polar media using a charge inducing agent and fabricating functional nanostructured thin films based on LbL assembly. This new LbL approach enables the incorporation of non-water soluble nanomaterials into thin films in their native state and eliminates extra processing steps that are necessary to make them water-soluble. Understanding fundamental aspects of electrostatic interactions in non-polar solvents will further extend the new LbL technique for the fabrication of multifunctional nanostructured thin films.
Emulsions and bubbles are multiphasic fluid mixtures that offer numerous applications in a wide range of areas from food and biomedicine to advanced materials. In this thrust, we use microfluidic techniques to generate engineered emulsions and bubbles that are used as templates to fabricate multifunctional suprastructures. In particular, we take exploit interfacial phenomena at the fluid interfaces to direct assembly of nano-/soft materials to fabricate functional materials for reactive separations, light-weight materials, ultrasound theranostics and drug delivery.
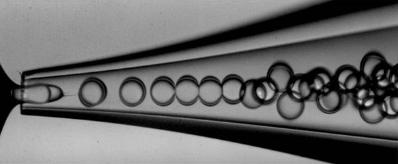
Projects
Hierarchical and asymmetric BIJEL microparticles, fibers and membranes.Bijels (bicontinuous interfacially jammed emulsion gels) are a new class of multiphasic mixtures that are formed by arresting the spinodal decomposition of immiscible liquids with interfacially jammed colloidal particles. In contrast to conventional emulsions, bijels have stable bicontinuous liquid architectures, making them ideal for applications that require intimate contact between two immiscible liquid phases and potentially mass transfer through the interface. Although bijels clearly present important new opportunities, the conventional preparation method is limited to privileged pairs of liquids that undergo temperature-induced phase separation, and cannot be widely adopted for other types of liquid pairs and for broad composition ranges. Furthermore, bijels prepared by this technique lose their stability when the temperature is changed and also tend to have features that are in the micron-scale range, limiting their interfacial area. Finally, these temperature quench methods have been performed in batch processes; a vessel filled with components is quenched to produce the bijel. To fully realize the promise of these unique bicontinuous liquid structures, it is highly desirable to develop methods that allow for continuous production of thermally stable bijels using a diverse pallet of liquids and particles. We recently presented the continuous generation of hierarchically and asymmetrically structured bijel fibers, microparticles and membranes based on solvent transfer-induced phase separation (STRIPS). In STRIPS, phase separation is induced by selective extraction of a solvent from an originally homogeneous mixture of three liquids. A homogeneous ternary liquid mixture comprising two immiscible liquids (typically, oil and water) and a solvent is introduced into a continuous phase that extracts the solvent from the ternary mixture to induce phase separation. The bicontinuous morphology that develops during STRIPS is stabilized by attachment of cationic surfactant-modified nanoparticles to the oil-water interface. The bijel fibers that we form using STRIPS have bicontinuous internal structures and are able to support the contact of immiscible fluids throughout their structures within which species can selectively migrate, which we demonstrated by the diffusion of hydrophobic (green) and hydrophilic (blue) dyes in the oil and water domains within the fiber, respectively.
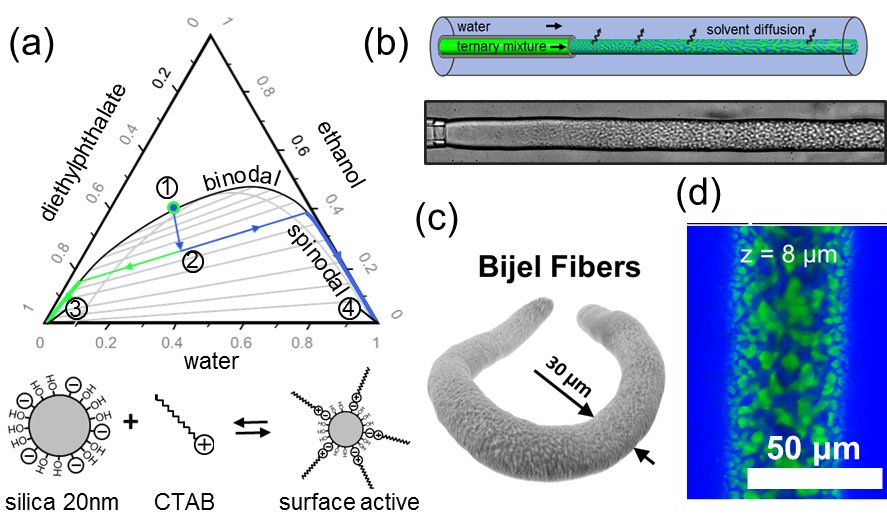
One-Step Formation of Smart Polyelectrolyte Microcapsules via Nanoscale Interfacial Complexation in Emulsions (NICE). Microcapsules that encapsulate and protect molecules and materials by forming isolated aqueous compartments inside hollow shells are widely used in a variety of applications in the food, pharmaceutical, cosmetics and agriculture industry. One promising method that has emerged in the recent years for the preparation of functional hollow microcapsules with aqueous cores involves layer-by-layer (LbL) assembly. Although the useful functionality of LbL microcapsules has been demonstrated, these successes have mostly been limited to laboratory/small-scale demonstration of such functionality. The widespread application of these LbL microcapsules in industry has not been fully realized. The major bottlenecks that have limited the widespread adoption of this promising technology are: 1) encapsulation efficiency is very low because this approach involves core dissolution and subsequent introduction of materials into the microcapsule lumen through the shell, and 2) although clever approaches to enhance encapsulation efficiency by using sacrificial porous particles have been developed, LbL assembly is nevertheless extremely tedious and time consuming. The objective of this effort is to investigate the processing-structure-property relationship of a new class of polyelectrolyte microcapsules with high encapsulation efficiency that are fabricated by a recently developed method based on the nanoscale interfacial complexation in emulsions (NICE). The proposed work is motivated by our recent success in one-step generation of water-in-water polyelectrolyte microcapsules with high encapsulation efficiency. The NICE method involves interfacial complexation of polymers at an oil-water interface in a multiphasic system such as double emulsions, followed by a spontaneous dewetting phenomenon. This method can be readily extended to systems using conventional multiple emulsions or single emulsions suspended over a macroscopic water-oil interface. The NICE microcapsules are potentially transformative because 1) their fabrication method is highly scalable, 2) the NICE method results in high encapsulation efficiency, 3) NICE capsules can encapsulate both hydrophilic and hydrophobic species in the core and shell of the microcapsules, respectively, and 4) microcapsules with functionality that rival that of LbL capsules can be generated.
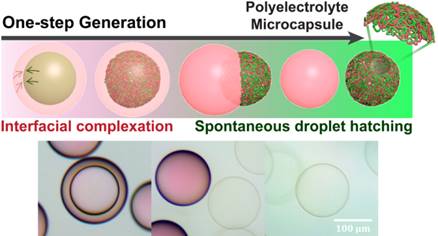
Recombinant Protein-Stabilized Microbubbles for Cancer Theranostics. There has been a significant interest in developing targeted therapies for cancer treatment. Our vision is to develop a radically new protocol to detect and treat cancer using microbubbles that combine the benefits of contrast-enhanced ultrasound imaging, microfluidics, antivascular ultrasound therapy (AVUST) and recombinant protein technology. This collaboration among the three research groups at Penn (Lee, Hammer and Sehgal (Penn Medical)) is motivated by our recent success in generating highly uniform microbubbles that are stabilized by recombinant protein (oleosin) which imparts endless possibilities in engineering the structure and properties of microbubbles for cancer theranostics. Our work has demonstrated that oleosin-stabilized microbubbles are highly monodisperse, stable and echogenic, making them ideal for AVUST applications. We are currently exploring methods to tune the mechanical properties of these oleosin-stabilized microbubbles and to impart targetting capabilities using recombinant protein technology.
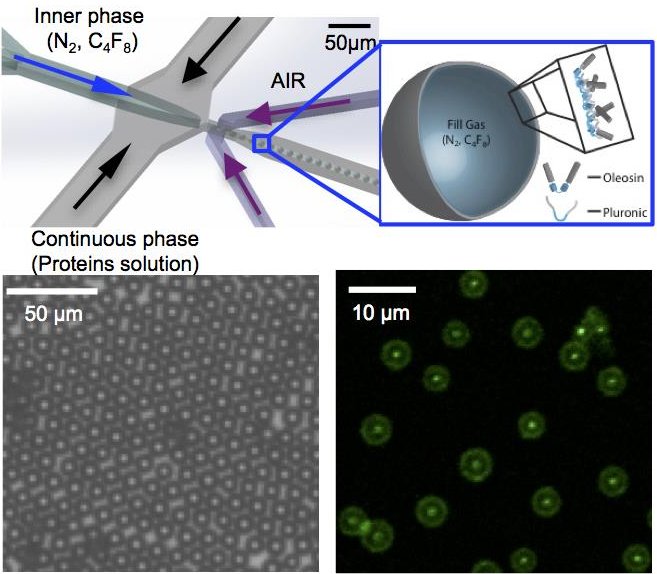
Scale-up of microfluidic emulsion production. Microfluidics enables the generation of highly uniform emulsions with unprecedented control over their morphology, which represents new opportunities to transform various fields including materials synthesis and high-throughput biological assays. However, the translation of droplet microfluidics technology into the commercial scale has been limited to a very few cases. One of the main reasons we believe such translation has not been so widespread is due to the low production rate of emulsions via microfluidic methods (< 1 mL/hr). Scale-up of droplet generation to the industrial (>1 L/h) scale is absolutely necessary to enable various fields to embrace the technology. To address this challenge, we have recently developed a three-dimensional monolithic elastomer device (3D MED) for mass production of monodisperse emulsion droplets. Using a method called double-sided imprinting, we can generate three dimensional microchannels a single elastomer piece that has 1000 parallel flow focusing generators. The 3D MED eliminates the needs for alignment and bonding of multiple layers to make three dimensional microfluidic channels and thus makes it possible to achieve the high flow rates and pressure necessary for the large-scale production of highly uniform droplets. Using this approach, we have achieved production rates as high as 1.5 L/h (>30 billion 45 µm diameter droplets per hour), with a coefficient of variation of droplet diameter of only 6.6%. Because of its simplicity and robusetness, 3D MED has the potential to play a major role in bridging the gap between the continuously growing library of promising microfluidic technologies and their successful translation in industry.
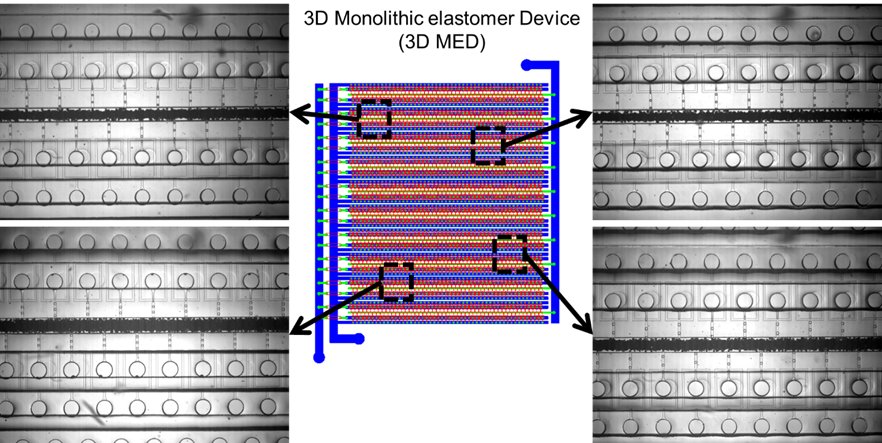
Bubble-Derived Light-Weight Materials. Enhancing the energy efficiency of land/sea/air transportation vehicles and equipment is one of the most challenging energy issues we face today. A very effective method to enhance the fuel efficiency of transportation vehicles is to reduce their body weight. While this idea sounds enticing, it is not straightforward to reduce the density of a material without sacrificing its mechanical strength and damage tolerance. Simply put, light-weight (i.e., low-density) materials tend to be weak. A major goal of our research program is to develop light-weight composites, also known as syntactic foams, with low density and high mechanical strength, toughness and durability. Such materials will have broad impacts in multiple industries including transportation, construction, furniture and electronics. Syntactic foams are composites made by incorporating hollow particles known as microballoons, cenospheres or bubbles into a metal, polymer or ceramic matrix. The incorporation of hollow filler particles lower the overall density of the composite, while its specific strength (strength divided by the material density) is enhanced. Our current focus is on understanding the processing-structure-property relationship of nanoparticle-shelled bubbles templated by microfluidic compound bubbles. We are using in situ quantitative techniques, nanoindentation and finite element analysis (FEA) to understand the effect of bubble structure on their mechanical behavior and fracture behavior.
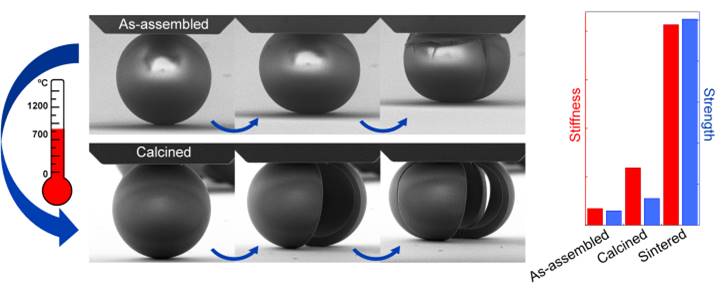
