Fundamental Atomistic Studies of Solid-State Aggregation
Our work in this area is concerned with the study
of basic mechanisms of diffusion, reaction, and aggregation in complex
environments such as external fields and compositional variations.
The aim is to be able to accurately describe these processes in
simulation tools. While molecular dynamics (MD) simulations only
require an interatomic force-field as input and therefore are able
to predict mechanisms, coarser approaches, such as continuum rate
equation (CRE) modeling and kinetic Monte Carlo (KMC), require that
all the relevant physical and chemical mechanisms be explicitly
specified a priori. Coarse-grain simulations are necessary
to access length and time scales of practical interest.
We have developed an “overlap” multiscale
computational framework in which molecular dynamics simualtions
are used to generate "experimental data" to which coarser
simulations can be fitted. The advantage of this approach is that
the MD-generated data comes from a material that is fully specified
- i.e. we know exactly all of its physical properties becuase they
can be computed using the same force-field used to generate the
experimental data! In this way, the only uncertainties in the coarse
model are mechanistic, which can be subsequently probed in detail
by the two-scale comparison. A schematic of this approach is shown
in Figure 1:
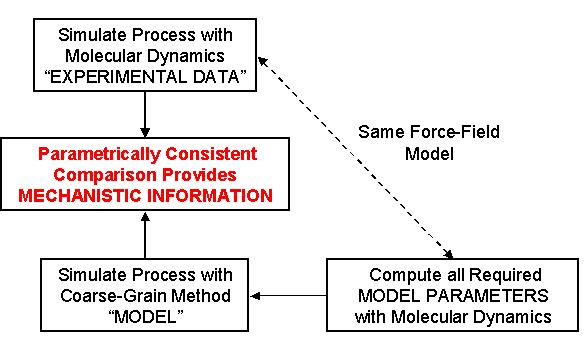
Figure 1: Multiscale overlap approach
for investigating nanoscale phenomena.
One application example is shown in Figure 2, which
demonstrates how a continuum model of vacancy aggregation in silicon
can be constructed to quantitatively reproduce
the dynamics predcited by a large-scale MD simulation, in which
1000 vacancies in a 216,000-atom lattice were allowed to evolve
under conditions of strong supersaturation. The parametrically consistent
comparison led to the discovery of previously ignored microscopic
physical phenomena such as surprisingly large cluster mobilities
and cluster shape fluctuations that dramatically increase the capture
radius for aggregation. In subsequent comparisons to experimental
data, these additional physical components were found to greatly
enhance the quantitative accuracy and predictive capability of continuum
models for void evolution during silicon crystal growth.
......................................... 
Figure 2: Parametrically consistent
comparison between MD and continuum model predictions for vacancy
aggregation.
|